The HL-LHC WP5 activities started in LS2 with some important upgrades in preparation to the full deployment of HL-LHC in LS3. The first phase of the collimation system upgrade during LS2 involved the installation of:
- 8 low-impedance secondary collimators in IR7;
- 4 low-impedance primary collimators in IR7;
- 2 dispersion suppressor collimators in the connection cryostats around IR2;
- 2 crystal primary collimators in IR7;
- 2 passive absorbers in IR7.
The updated LHC collimation system layout for the recently started Run 3 is shown in Figure 1. Primary collimators and passive absorbers are part of the consolidation of the collimation system that the HL-LHC will profit from, as they will remain installed for the entire HL-LHC lifetime. Together with spare collimator units, and with the dispersion suppressor collimators built for IR7 (whose installation was deferred following the decision not to install the 11 T dipoles during LS2), a total of 25 new devices were successfully produced and validated for operation! The installation in the LHC tunnel proceeded well, an example showing a crystal collimator being transported along IR7 can be seen in Figure 2.
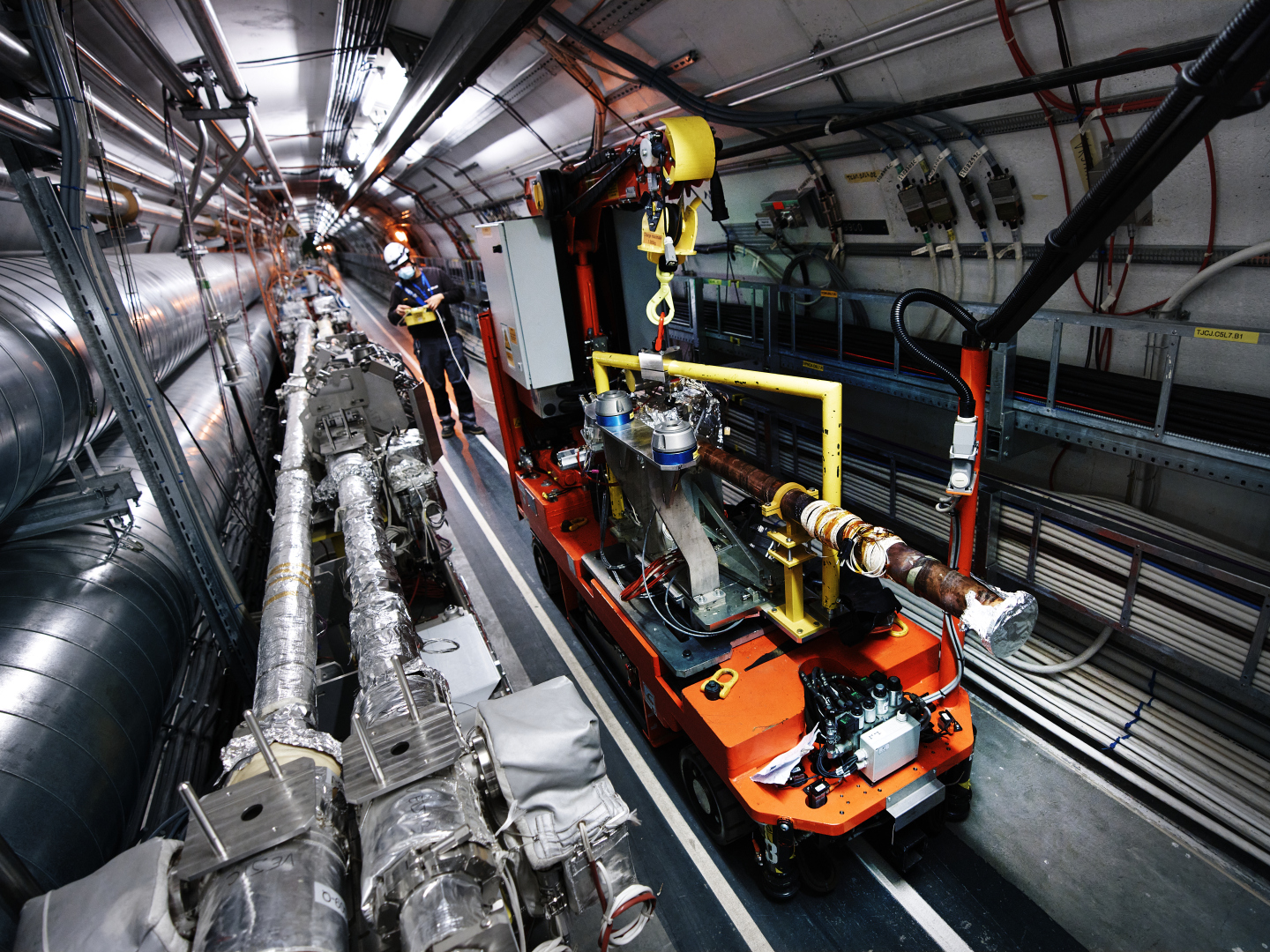
This first phase of the upgrade is on the one hand motivated by the need to fulfill the goals for the operation of the LHC as a heavy-ion collider. The Pb beam current will nearly double, and the ALICE peak luminosity will be ~6 times higher than in Run 2. This requires improvements of the IR7 cleaning and of the IR2 collimation, respectively. On the other hand, the important reduction of the IR7 impedance will allow pushing the proton beam performance well beyond the bunch currents used in Run 2.
All the new HL-LHC collimators were successfully installed during LS2 and put in operation already for the LHC pilot-beam run in 2021, except for the crystal collimators that were installed just after the beam tests (Nov. 2021). All new collimators are now being commissioned for the 2022 run at high intensity. The jaw movement controls are fully integrated in the collimation control system: collimators can be aligned in the same way as the “old” system, using the machine learning algorithms developed in Run 2. The HL-LHC collimators feature in addition in-jaw beam position monitors for even faster alignment and orbit monitoring throughout the cycle, which will be used for an early detection of possible unsafe conditions in case of orbit shifts.
The new crystal primary collimators have also been tested with beam: both new devices were aligned at 450 GeV, showing the expected halo-channeling properties (see an example in Figure 3). Beam tests need to continue, in particular to complete the setup at 6.8 TeV, to prepare them fully for the operation with lead ion beams planned at the end of the 2022 run. This will be the first time that crystal channeling will be used as main collimation method for regular high-intensity physics operation, which is an important milestone.
Altogether, the successful start of beam operation demonstrated that the newly installed and upgraded collimators behave as expected and are all set for a successful intensity ramp up for the 2022 run, both for proton and ion beams.